In this blog, we will address and explain the importance of developing maximal strength, rate of force development, torque and reactive strength during the rehabilitation process for athletes following a sports injury.
Maximum Strength
The development of muscle strength can be broadly divided into morphological and neural factors (1). The maximum strength produced by a single muscle fiber is directly proportional to its cross-sectional area, which is determined by the number of sarcomeres in parallel, an important parameter in the ability to generate force (2, 3).
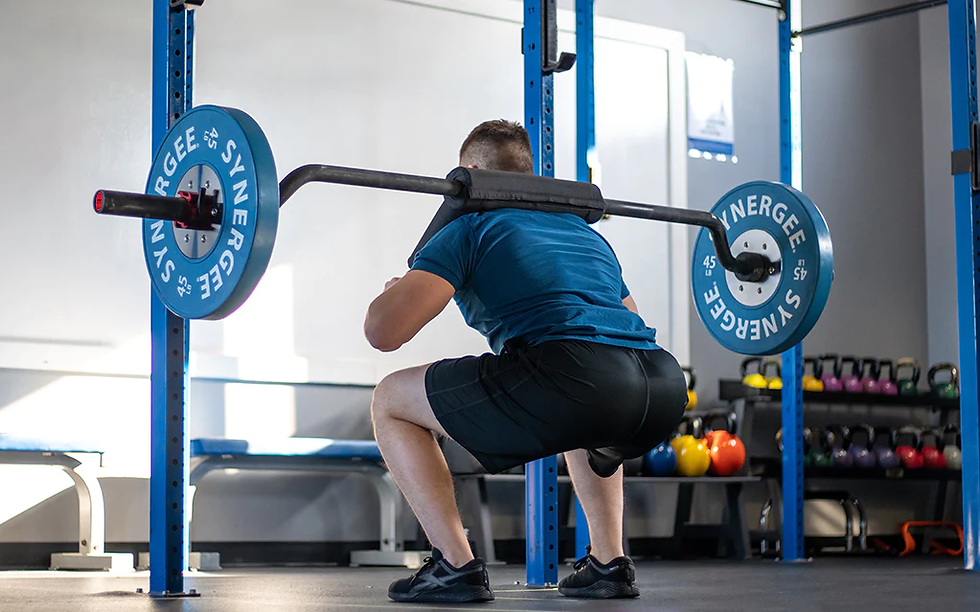
Greater penetration angles are more common in hypertrophied muscles than in normal muscles. Maximum strength is also influenced by the composition of muscle fibers (1, 4, 5). Specially, type II fibers have a greater capacity to generate energy per unit of cross-sectional area than type I fibers. Architectural characteristics, such as longer beam length, allow for greater force production through an optimal length-tension relationship (1).
In sports, the ability to generate maximum force is limited by the time constraints of specific task. Thus, the rate of force development (RFD) and power are critical aspects of optimizing sports performance. Maximum strength can be defined as the upper limit of the neuromuscular system to produce force (6), with increases in this capacity correlated with RFD and power (7, 8).
Current evidence suggests that until athletes can squat with at least 1.6 times their body weight, maximum strength training should be the dominant training modality (9).
The development of maximum strength demonstrates significant benefits in musculotendinous stiffness (10), neuromuscular inhibition (4) and connective tissue strength (11), resulting in decreases in relative force applied during the loading phase in ground contact (12). Collectively, this reduces the metabolic demand for the same force output, creating an additional pool of motor units available for work (12). In addition to the physiological and performance advantages of maximum strength development, it is not surprising that the risk of injury may be reduced with the adoption of this training modality (13).
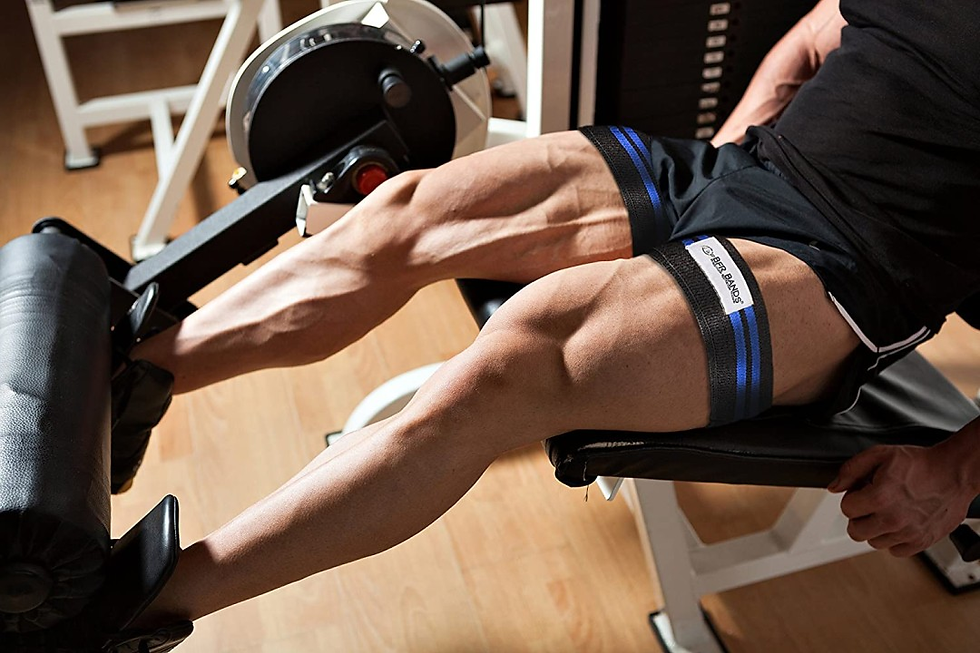
The literature indicates that the prescription of maximum strength training should involve a load (or intensity) of 80-100% of the participant’s one-repetition maximum (1RM), using approximately 1-6 repetitions, in 3-5 sets, with rest periods of 3-5 minutes and a frequency of 2-3 times per week (14). Consequently, for physiotherapists whose specific goal in a particular phase is to improve maximum strength, they should progressively work toward this load and volume prescription (13).
In the early stages, when athletes may not have the capacity to tolerate high loads, lower intensities in various high-volume sets to momentary failure can be employed to recruit higher threshold motor units and increase cross-sectional area (15, 16). Alternatively, blood flow restriction training can be used to provide an effective stimulus during rehabilitation in athletes with impaired ability to tolerate high loads (17).
In addition to the above, a “cross-education” phenomenon (high-resistance training in the unaffected limb) may also be a viable option to reduce corticospinal inhibition, increase strength in the contralateral limb and induce hypoalgesia (18 – 20).
A potencial progression based on the rehabilitation phase and post-surgical irritability of the anterior cruciate ligament patient may be (13):
Unipodal bodyweight squat performed in high-volume sets with a focus on technique and cross-education.
Unipodal squat with light load and high-volume sets to failure (with or without blood flow restriction).
Split squat with progressive load in a traditional periodization scheme until reaching the recommended condition for maximum strength.
Split squat performed according to maximum strength recommendations, with possible adaptations outlined in the figure below.
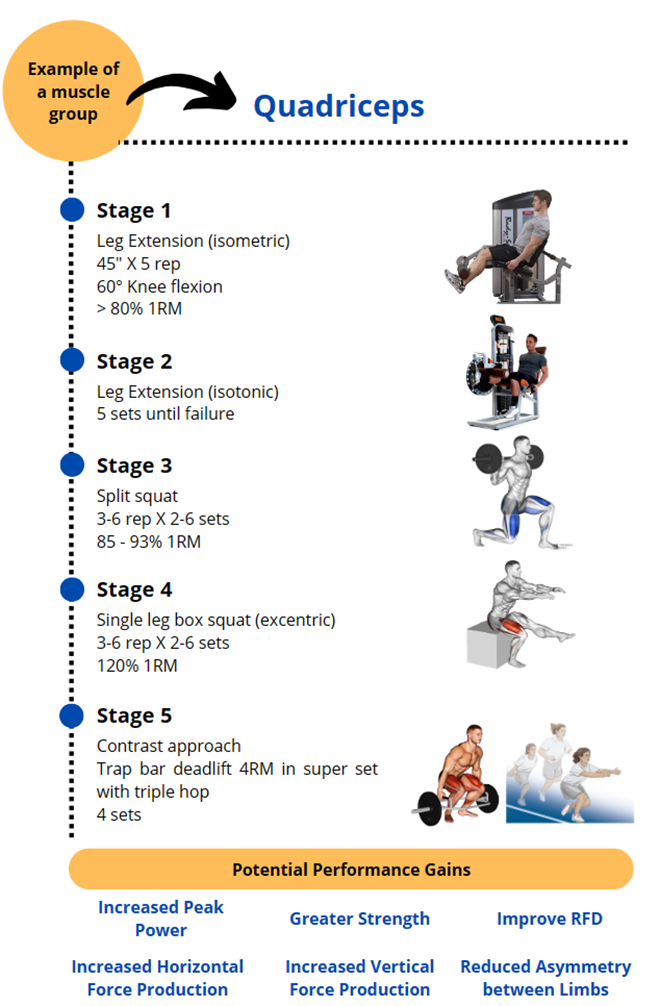
From a rehabilitation perspective, isometric contractions can be used during specific phases when dynamic contractions are contraindicated. Although dependent on the musculoskeletal condition, isometric contractions are capable of inducing hypoalgesia in some injuries. However, the hypoalgesic effect is variable and not always consistent (21, 22).
During isometric contractions, the muscle-tendon unit remains at a constant length. Isometric muscle actions have been widely used due to their tightly controlled application of force at specific joint angles, their ability to generate greater force than concentric contractions, and their high reliability in assessing and tracking force production (23).
Rate of Force Development (RFD) and Torque
RFD is defined as the ability of the neuromuscular system to produce a high rate of increase in muscle force per unit of time during the initial phase and after the onset of contraction (7). On the other hand, torque refers to a force that causes rotation. Contractile RFD is a parameter used to measure “explosive” strength capabilities (13).
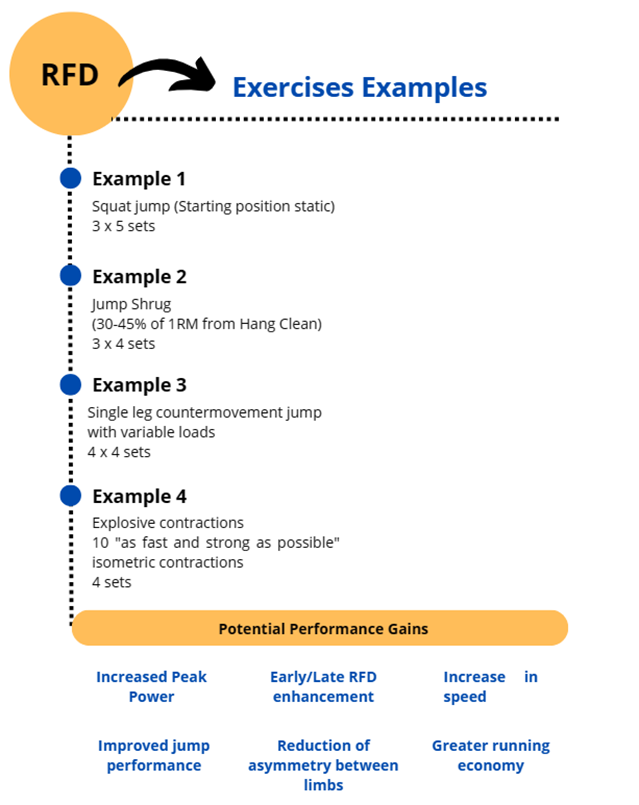
Contractile RFD is determined from the slope of the force/time curve (usually between 0 and 250 ms) and calculated as Force/Time. Several factors can impact RFD, particularly the initial phase ( <100 ms from the onset of contraction), which is more influenced by intrinsic muscle properties and neural drive, while the late phase ( >100 ms from the onset of contraction) responds more to maximum muscle force (7).
Considering that force application during tasks such as running, jumping and striking lasts approximately 30-200 ms (8), RFD is a critical performance characteristic central to success in most power-based sports as well as running performance (24).
Current evidence indicates that training at high speeds or with the intention of moving loads quickly is highly effective in producing rapid force (25, 26). In the figure at the right, we can observe some examples of exercises.
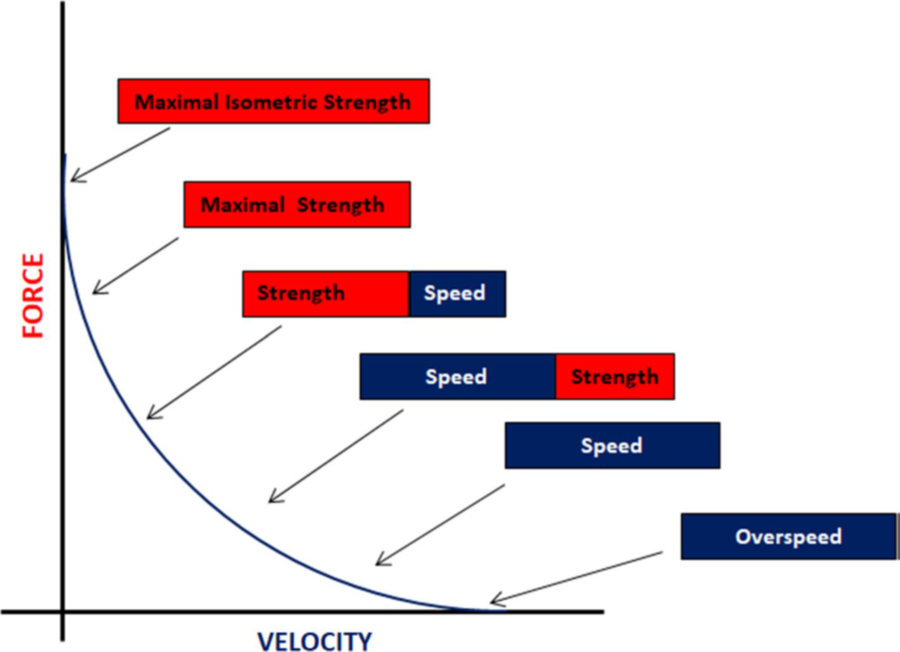
The prescription of these exercises can be better appreciated by defining the parameters that underpin power. Mechanically, power is the work done per unit of time, or force multiplied by velocity. The inverse relationship between force and velocity can be illustrated through the force/velocity curve, which identifies that maximum force is exerted under high loads, and maximum velocity is produced under low loads (8).
Subsequently, the goal of strength and conditioning programming is to enhance strength capacity across the entire spectrum of loads and, therefore, velocities (13).
Reactive Strength
Eccentric actions are those in which the musculotendinous unit undergoes active elongation along the muscle action. Eccentric training includes superior benefits in concentric and isometric force production, preferential recruitment of type II muscle fibers, power, RFD, stiffness, muscular architecture, increased muscle activation, as well as improved performance in sports actions (4).

Strong eccentric contractions can have a superior impact on reducing inhibition and increasing intracortical facilitation (18). These improvements can occur where there are high loads of eccentric stretching, such as in jump landings, directional changes and fast stretch-shortening cycles (SSC), as an athlete’s reactive strength is sustained by relative maximum eccentric strength (27). These facts reinforce the need for substantial levels of strength values before developing SSC capabilities (28).
The Reactive Strength Index (RSI) has been widely used to quantify plyometric or SCC performance, i.e., the ability to rapidly transition from an eccentric to a concentric muscle action (29). Factors supporting efficient SSC are related to the storage and reuse of elastic energy. These results from a series of mechanisms, including the use of intrinsic muscle-tendon stiffness, involuntary reflex muscle activity, antagonist co-contraction and pre-stretching of the SSC (30).
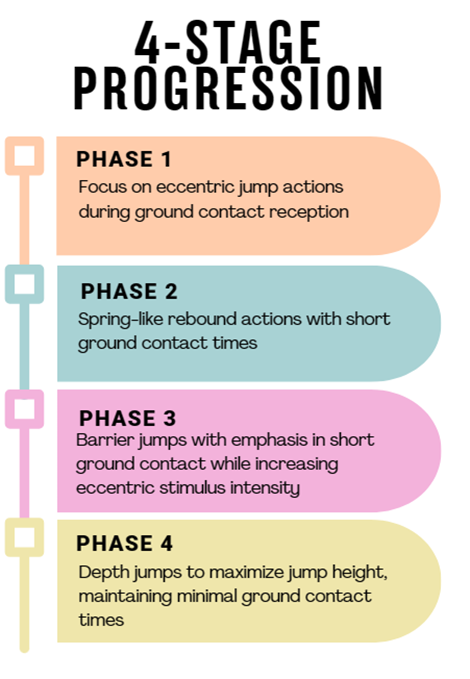
RSI can be used to assess lower limb stiffness, described as the resistance to deformation of the limb in response to force application. Therefore, a certain amount of lower limb stiffness is required for effective storage and reuse of elastic energy in SSC activities (24). Lower limb stiffness is considered as key attribute in enhancing running, jumping and activities such as hopping (31).
Achieving an adequate level of strength is crucial for the development of reactive strength, as discussed earlier. Plyometric training explores the rapid cyclical muscle action of the SSC, where the muscle undergoes a stretching movement (eccentric muscle action), followed by a transition period before the shortening movement (concentric contraction) and can be used to enhance eccentric force production capacity (13).
Flanagan et al. (29) suggest a 4-stage progression as you can see on the figure.
Bibliographic References
1. Cormie P, McGuigan MR, Newton RU. Developing maximal neuromuscular power: part 1—biological basis of maximal power production. Sports Med. 2011;41(1):17–38.
2. Hornsby WG, et al. What is the impact of muscle hypertrophy on strength and sport performance? Strength Cond J. 2018;40(6):99–111.
3. Taber CB, et al. Exercise-induced myofibrillar hypertrophy is a contributory cause of gains in muscle strength. Sports Med. 2019;49(7):993–7.
4. Suchomel TJ, et al. The importance of muscular strength: training considerations. Sports Med. 2018;48:765.
5. Hughes DC, Ellefsen S, Baar K. Adaptations to endurance and strength training. Cold Spring EHarb Perspect Med. 2017;8(6).
6. Stone MH, et al. The importance of isometric maximum strength and peak rate-of-force development in sprint cycling. J Strength Cond Res. 2004;18(4):878–84.
7. Rodríguez-Rosell D, et al. Physiological and methodological aspects of rate of force development assessment in human skeletal muscle. Clin Physiol Funct Imaging. 2018;38(5):743–62.
8. Taber C, et al. Roles of maximal strength and rate of force development in maximizing muscular power. Strength Cond Res. 2016;38(1):71–8.
9. Cormie P, McGuigan MR, Newton RU. Adaptations in athletic performance after ballistic power versus strength training. Med Sci Sports Exerc. 2010;42(8):1582–98.
10. Bohm S, Mersmann F, Arampatzis A. Human tendon adaptation in response to mechanical loading: a systematic review and metaanalysis of exercise intervention studies on healthy adults. Sports Med Open. 2015;1(1):7.
11. Magnusson SP, Kjaer M. The impact of loading, unloading, ageing and injury on the human tendon. J Physiol. 2019;597(5):1283–98.
12. Stone M, Stone M, Sands W. Principles and practice or resistance training. New York: Human Kinetics; 2007.
13. Maestroni L, Read P, Bishop C, Turner A. Strength and Power Training in Rehabilitation: Underpinning Principles and Practical Strategies to Return Athletes to High Performance. Sports Med. 2020 Feb;50(2):239-252.
14. American College of Sports Medicine position stand. Progression models in resistance training for healthy adults. Med Sci Sports Exerc. 2009;41(3):687–708.
15. Schoenfeld BJ, et al. Strength and hypertrophy adaptations between low- vs high-load resistance training: a systematic review and meta-analysis. J Strength Cond Res. 2017;31(12):3508–23.
16. Schoenfeld BJ, Grgic J, Krieger J. How many times per week should a muscle be trained to maximize muscle hypertrophy? A systematic review and meta-analysis of studies examining the effects of resistance training frequency. J Sports Sci. 2018;37:1–10.
17. Hughes L, et al. Blood flow restriction training in clinical musculoskeletal rehabilitation: a systematic review and meta-analysis. Br J Sports Med. 2017;51(13):1003.
18. Kidgell DJ, et al. Increased cross-education of muscle strength and reduced corticospinal inhibition following eccentric strength training. Neuroscience. 2015;300:566–75.
19. Cirer-Sastre R, Beltrán-Garrido JV, Corbi F. Contralateral effect after unilateral strength training: a meta-analysis comparing training loads. J Sports Sci Med. 2017;16(2):180–6.
20. Vaegter HB. Exercising non-painful muscles can induce hypoalgesia in individuals with chronic pain. Scand J Pain. 2017;15:60–1.
21. O’Neill S, et al. Acute sensory and motor response to 45-s heavy isometric holds for the plantar flexors in patients wit Achilles tendinopathy. Knee Surg Sports Traumatol Arthrosc. 2018;27(9):2765–73.
22. Riel H, Vicenzino B. The effect of isometric exercise on pain in individuals with plantar fasciopathy: a randomized crossover trial. Scand J Med Sci Sports. 2018;28(12):2643–50.
23. Oranchuk DJ, et al. Isometric training and long-term adaptations; effects of muscle length, intensity and intent: a systematic review. Scand J Med Sci Sports. 2019;29(4):484–503.
24. Brazier J, et al. Lower extremity stiffness: considerations for testing, performance enhancement, and injury risk. J Strength Cond Res. 2019;33(4):1156–66.
25. Maffiuletti NA, et al. Rate of force development: physiological and methodological considerations. Eur J Appl Physiol. 2016;116(6):1091–116.
26. Tillin NA, Pain MT, Folland JP. Short-term training for explosive strength causes neural and mechanical adaptations. Exp Physiol. 2012;97(5):630–41.
27. Beattie K, et al. The relationship between maximal strength and reactive strength. Int J Sports Physiol Perform. 2017;12(4):548–53.
28. James LP, et al. The impact of strength level on adaptations to combined weightlifting, plyometric, and ballistic training. Scand J Med Sci Sports. 2018;28(5):1494–505.
29. Flanagan EP, Comyns TM. The use of contact time and the reactive strength index to optimize fast stretch-shortening cycle training. Strength Cond J. 2008;30(5):32–8.
30. Pedley JS, et al. Drop jump: a technical model for scientific application. Strength Cond J. 2017;39(5):36–44.
Lum D, et al. Effects of intermittent sprint and plyometric training on endurance running performance. J Sport Health Sci. 2019;8(5):471–7.
Comentarios